2 Bragg Crystal Spectrometer (BCS)
2.1 Instrument Characteristics
2.1.1 Wavelength Range
2.1.2 Data Compression
2.2 Instrument Calibration
2.2.1 Sensitivity Functions
2.2.2 Wavelength Resolution
2.3 Detector Performance
2.3.1 Detector Gain and Energy Resolution
2.3.2 Position Resolution
2.3.3 Digitization Spikes
2.4 Detector Background
2.4.1 Energy Discrimination
2.4.2 Particle Background
2.4.3 Germanium Fluorescence
2.4.4 Effect of Gain Depression
2.5 Dynamic Range and Count Rate Effects
2.5.1 Deadtime Correction
2.5.2 Saturation
2.5.3 Gain Depression
2.5.4 Rate-dependent Distortion
2.6 Data Features
2.6.1 Queued Data
2.6.2 Timing of Spectra
2.6.3 Last Spectral Bin
2.6.4 Medium-rate Spectral Data in Autumn 1991
2.6.5 Single Event Upsets
2.7 Contact Persons
2.8 References
2 Bragg Crystal Spectrometer (BCS)
The Bragg Crystal Spectrometer (BCS) is designed to study plasma
heating and dynamics during the impulsive phase of solar flares. It
consists of two bent crystal spectrometers, BCS-A and BCS-B, that
observe the H-like line complex of Fe XXVI, and He-like complexes of
Fe XXV, Ca XIX and S XV. Each spectrometer consists of a double
detector placed behind a pair of germanium crystals that diffract the
incoming X-rays into the detectors. The two structures are mounted at
the front of the Yohkoh spacecraft, on either side of the central
panel, with a thermal filter mounted in front of each spectrometer.
The crystal dispersion axes are oriented north-south.
There is much more about the performance of the BCS instrument than is
reported here. If additional information on the design and
performance of the BCS is needed, the user is encouraged to consult
the documents listed in Section 2.8.
2.1 Instrument Characteristics
2.1.1 Wavelength Range
The designed and laboratory measured wavelength ranges are given in
the table below:
Chan Designed Wavelength Bin Range Lab. Measured Wavelength
1 1.7636 -- 1.8044 212 -- 28 1.7597 -- 1.8121
2 1.8298 -- 1.8942 224 -- 36 1.8284 -- 1.8957
3 3.1631 -- 3.1912 27 -- 229 3.1633 -- 3.1933
4 5.0160 -- 5.1143 40 -- 234 5.0163 -- 5.1143
It should be stressed that these wavelengths are only valid at the
designed, nominal BCS boresight. Because of the nature of the
way a bent crystal spectrometer works, if a source is observed at
other than the boresight then the wavelength range observed by the
detector is shifted. In order to allow the SXT to observe more
of the northern polar corona, the Yohkoh spacecraft is normally
pointed several arcmins north of its designed pointing; because the
dispersion axes of the crystals are oriented in the north-south
direction, with this offset pointing channels 1 and 2 are normally
seeing wavelengths that are slightly shorter than designed, and
channels 3 and 4 slightly longer than designed. Consequently, during
times of the year when the north pole of the Sun is tilted towards the
Earth (i.e. Oct-Jan), if the active region is in the southern solar
hemisphere, then this offset pointing severely compromises the ability
of the BCS to observe the blue-wing in Ca XIX (channel 3).
The bin shifts caused by an offset in the pointing were measured
in the laboratory to be (6.634±0.039) 10-2 bins/arcsec,
(4.730±0.025) 10-2 bins/arcsec, (8.218±0.062) 10-2
bins/arcsec and (4.305±0.028) 10-2 bins/arcsec for channels 1
to 4 respectively (note: these are per single bin - channels 3 and 4
are often double binned). These values are being confirmed by in-orbit
measurements which compare the bin of the resonance line with the
source position observed by SXT. The above values correspond to
15.074, 21.142, 12.168 and 23.229 arcsec/bin.
2.1.2 Data Compression
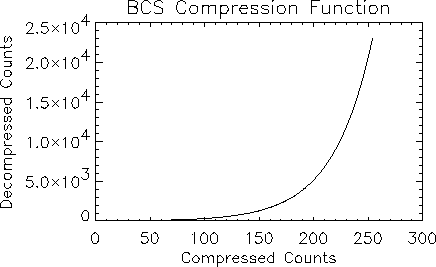
Figure 2.1:
The BCS data compression algorithm
All the BCS spectral data are output to telemetry as compressed
counts. The compression is done by a hardware lookup table held in ROM (read-only memory)
using a compression scheme that minimizes the errors. It is a
complex function and is shown in Fig 2.1.
2.2 Instrument Calibration
The BCS instrument was calibrated at the Rutherford Appleton
Laboratory (RAL). This work is described in Lang et al., 1993.
2.2.1 Sensitivity Functions
The response of a spectrometer channel depends on the transmission
of the detector window (as a function of its length), the detector
linearity, the crystal curvature and the transmission of the thermal
filter. All three of these factors were independently measured during
construction of the BCS and an all-up check was then performed at
RAL. The response files for each of the channels are in the
directory defined by ``$DIR_BCS_CAL'' under the names BCSA1.nnn,
BCSA2.nnn, BCSB3.nnn and BCSB4.nnn, where nnn is the file version number.
The sensitivity functions contained in these files are
listed in tables in an appendix of Lang et al., 1993.
These functions represent the departure from the nominal efficiency,
or effective area of the spectrometers. The measured effective areas,
with uncertainties, for the flight wavelength ranges are
0.104±0.009 cm2, 0.114±0.012 cm2, 0.303±0.032
cm2 and 0.071±0.010 cm2 for channels 1 to 4 respectively
when the relative response within a channel is taken to be uniform.
2.2.2 Wavelength Resolution
The wavelength resolution for a particular BCS channel depends on
the rocking curve of the crystal for that channel, on the position
resolution of the detector, and on the angle that the photons enter
the detector in that channel. The latter point is more relevant to the
higher energy photons of channels 1 and 2 - these photons penetrate
further into the detector volume and if their path is not
perpendicular (to the window) they will produce an electron cloud that
spans a longer length of the wedge-and-wedge cathode, and hence a
broader positional distribution.
2.3 Detector Performance
2.3.1 Detector Gain and Energy Resolution
The gain and energy resolution of the detectors are dependent on the
gas mixture and the high-voltage (HV) setting. The detector
performance is regularly checked using a Fe55 radioactive source.
There are 8 setting of the high voltage at 30 volt intervals that can
be used to compensate for changes in the detector gain due to
contamination (gain decreases) or leakage (gain increases) - each
step represents a change of a factor of ~1.28 in gain. The trims
on both HV units were set to the nominal value of 4 (1476 V) at
launch. Although both detectors are showing a small, steady decrease
in gain (1.95% p.a. in BCS-A and 0.92% p.a. in BCS-B), it has not
yet been necessary (Jan/94) to change the HV trim setting. The history
of the HV trim settings are contained in file
``$DIR_BCS_LOGS/hv_log.dat'' .
During the radioactive source calibration, in order to make it
possible to detect the 5.9 KeV photons emitted by the Fe55
source, the gain of the detector for BCS-B is changed by setting an HV
trim level of 2 (1418 V).
The results of the PHA (pulse-height analyzed) pre- and post-launch detector calibrations performed
with a radioactive source are contained in the file
``$DIR_BCS_LOGS/gain_log.dat.''
A history of the the gain and energy resolution of the two BCS
detectors can be obtained by typing the IDL command:
IDL > .run gain_plot4
The variation of the gain with temperature (determined from measurements
made during the pre-launch Thermal Test) can be obtained by typing the
IDL command:
IDL > .run gain_temp_plot
2.3.2 Position Resolution
The position resolution of channels 1 and 2 are approximately 350 mm, and
channels 3 and 4 somewhat larger.
The wedge-and-wedge pattern of the detector can be stimulated through
a set of three pads located on the rear of the quartz plate. When a signal
is applied to a pad, it induces a charge on the pattern in the same way
that an incoming photon would, but at a known location. During a stim
test, each pad is stimulated in turn.
The position resolution and linearity of the detectors are regularly
measured using these electronic stims.
2.3.3 Digitization Spikes
The position of a photon in the detector is calculated from the
signals measured on the two wedges (Wa and Wb) of the
wedge-and-wedge cathode. The position is given by the formula:
x = [(Wa)/( Wa + Wb)]
In the BCS this calculation is done using a look-up table held in ROM.
Because of the quantized nature of this digital technique, solutions
sometimes fall either side of the value that would have been
determined with an analogue technique and spikes and dips are seen in
what should be a flat field. The position of these can be calculated,
and corrected - there is some dependence on the energy of the detected
photon. No photons are lost, they are merely displaced into adjacent
bins so integrations under curves are not affected, but when fitting
spectra it is best to avoid those bins.
2.4 Detector Background
2.4.1 Energy Discrimination
To allow the rejection of photons whose energies lie
outside the desired range, a pair of adjustable energy discriminators
(single channel analyzers, or SCA's) are provided for each channel of the BCS.
The history of the SCA settings are contained in file
``$DIR_BCS_LOGS/sca_log.dat''.
2.4.2 Particle Background
The BCS detectors are effected by a background caused by particles
trapped in the Earth's magnetic field. Many of these are rejected by
the setting of the lower level SCA, but occasionally some are seen in
channels 1 and 2 - they appear as a flare-like spike, but are not
seen at the corresponding time in channels 3 and 4.
Over the South Atlantic Anomaly, the background caused by trapped particles
is particularly intense and the High Voltage supplies to the BCS
detectors are switched off (using time-tagged commands).
2.4.3 Germanium Fluorescence
When germanium is illuminated by photons whose energy exceeds 11.2
keV, the crystal fluoresces, emitting a photon whose energy is 9.9
keV. Since the energy resolution of the BCS detectors is around 20%,
the tail of the distribution of these fluorescent photons falls within
the energy range that the BCS is sensitive to and indeed part of the
tail lies under the distribution of the photons detected by channels 1
and 2. In order to try to reject the fluorescence, the upper level
discriminator is set midway between the two energy distributions.
germanium fluorescence is less of a problem in channels 3 and 4 since
there is a greater difference in the energy between the channel
photons and the fluorescent photons.
2.4.4 Effect of Gain Depression
When the observed count rate increases the gain of the detector
becomes depressed and the position that the photons from a given
channel are recorded at (in SCA space) slips to lower values. As a
consequence, the perceived energy distribution of the photons can
move relative to the energy window defined by the lower and upper
level discriminators and some photons may be rejected as being out of
limits. This effect has made it difficult to reject the photons caused
by Ge fluorescence when the count rates are high.
2.5 Dynamic Range and Count Rate Effects
The dynamic range of a proportional counter of the type used on the
BCS is limited and it is not possible to observe both very weak and
very intense flares.
The BCS on Yohkoh was designed to have high sensitivity so that it can
observe as early as possible at the onset of a flare and the dynamic
range is therefore biased towards the smaller flares. As a consequence
the BCS saturates during larger flares, i.e at high count rates. During
saturation all positional information is lost but the total event
counter may still be valid.
2.5.1 Deadtime Correction
All analogue signals produced by the detectors take a time to
process that is significant when count rates are high. Although the
anode signals only take 1 ms to process, the processing of the
wedge signals takes 3.5 ms and additional time needs to be allowed
for the signal levels to settle back to zero so that in total 35 ms
must elapse between successive photons. Consequently, during this
``deadtime'' the processing of any new position signals is inhibited.
At low count rates this effect is small, but it becomes more important
as the count rate increases. If the photon arrival times were
evenly spaced, then the pair of channels in one detector could see a
maximum of ~28×104 counts/sec. However, in practice the
random nature of the events, and the onset of saturation, results in a
maximum of less than half this.
In the BCS there are three sets of counters for each channel: the
total event counter, the limited (or in-window) event counter and the
encoded event counter. The gate for the limited event counter only
accepts events that fall within the allowed energy window, and in
addition inhibits any event that arrives less than 35 ms after
the previous one. From a knowledge of the event counters, it is
possible to calculate the effects of the deadtime and hence
reconstruct what the count rate should be. This is done within the
MK_BSC and MKBSD routines.
It should be noted that while the event counters used for deadtime
corrections are in the DP-synchronous (DP_SYNC) part the telemetry, the
spectral data are in the PH stream and after passing through the queue
memory are asynchronous. As a consequence, if there are holes in the
data coverage, it is possible for the DP_SYNC data to be absent at
the time of the spectral observations. At such times it is not
possible to make a deadtime correction.
2.5.2 Saturation
The problem of saturation is exacerbated by the double detectors
used on the BCS. During construction, constraints on mass and volume
required that the two channels on a spectrometer shared the same
detector gas volume. Although the anode signals from the detectors
(which allow energy discrimination) are processed separately for each
channel, the positional information is provided by a wedge-and-wedge
pattern that is shared by the channels. Thus, the processing deadtime
for position encoding for a detector is affected by the sum of the
total count rates for both channels of that detector.
That the onset of saturation is determined by the counts in two
channels can produce ``interesting'' effects. For instance, if the flare
is hot, the detector of spectrometer BCS-B will saturate at a higher
count rate in Ca XIX (channel 3) than if it is cool. Normally the
count rate in S XV (channel 4) will dominate in BCS-B, but for a hot
flare the saturation will depend more on the Ca XIX than on the S XV
count rate.
In spectrometer BCS-A, Fe XXVI (channel 1) has only been observed in a
few flares because often the count rate in Fe XXV (channel 2) is already
very high by the time that Fe XXVI is likely to be observed.
It should be noted that adjusting the SCA values does not affect the
onset of saturation. The same number of photons are still entering the
detector and each is seen by the positional encoding circuitry no
matter how many are gated as having valid energies.
2.5.3 Gain Depression
As mentioned under the ``Detector Background'' section, when the
count rate is high, the gain of the detector becomes depressed and the
perceived energy distribution slides down in the SCA space, and
possibly partly or totally out of the energy window set by the SCAs.
As a consequence, the number of photons accepted as having valid
energy is reduced and since this signal is used to determine whether
the decoded position should be used, the number of encoded photons is
reduced.
2.5.4 Rate-dependent Distortion
When a large number of counts are put into a small length of the
detector volume, a space charge effect develops which attracts the
electron cloud towards that region of the detector. This results in
lines that are taller and narrower than they should be and locally
distorts the linearity of the detector.
This effect is not confined to spectral lines and can occur in any
region of the detector where the photon flux is large; the total
number of counts is conserved, but there is a loss of positional
information.
It is not clear that this effect can be deconvolved, and the matter is
still being studied. More information is given in Trow, Bento and
Smith (1993).
2.6 Data Features
2.6.1 Queued Data
The BCS spectral data are stored in a queue memory prior to
insertion in the telemetry frame. These data are therefore asynchronous
to the data in the DP synchronous area of telemetry and occasionally
the DP_SYNC data are not present at the same times as the spectral
data. During these periods, deadtime correction to the spectral data are
not possible. Key items relating to the mode being executed by the BCS
are included in header information included in the queued data and it
is always possible to know what mode the BCS is in.
At times a mode called ``Fast Queue'' is used. In this the data
accumulators are sampled at intervals without clearing and then the
data are stored in two different queue - normal and fast. The final
integration before clearing of the accumulator is stored in the normal
queue and all others are stored in the fast queue. In this mode, if
the flare-flag is raised the fast queue is dumped to telemetry, but
normally only data from the normal queue goes into telemetry. The mode
is used at times when the spacecraft is in a mode whose bit-rate does
not allow as short an accumulation time as is desired. The fast queue
allows high cadence data to be stored and only output if a flare
switches the spacecraft to a higher bit-rate.
Fast Queue data can be recognized by a sawtooth-type ramping in the
light curve. These type of data are normalized by the IDL function
BCS_NORM.
2.6.2 Timing of Spectra
The time contained in a mode record for a BCS spectrum represents
the start of the integration time of that spectrum. Thus a correction
(equal to half the integration period) needs to be added to the points
in a light curves for all plots that are NOT in histogram mode
(psym=10). The routine BCS_TVEC does this.
2.6.3 Last Spectral Bin
The last bin in each channel is used to accumulate positionally
encoded counts when the sum of the signals on the two wedge, or the
signal on any individual wedge is very small or very large. Although
useful as a diagnostic, these bins should ignored for analysis purposes.
2.6.4 Medium-rate Spectral Data in Autumn 1991
Just after the launch of Yohkoh, it was found that there was an
error in the way the DP inserted BCS PH-data into the telemetry frame
during medium bit-rate. This can be seen a ``hole'' that steadily marches
through the data in successive spectral integrations; when viewing the
light curve, a periodic, but structureless reduction in the count rate
is seen in medium bit rate. When the hole is not in an interesting part
of the spectrum, the data can be used, otherwise it should be
rejected. The problem was corrected by a patch to the DP in late
November 1991.
2.6.5 Single Event Upsets
At intervals, and particularly when the spacecraft has its apogee
over the South Atlantic, the memory of the BCS microprocessor can be
corrupted by a Single Event Upset (SEU). Often this affects a part of
the memory that does not disturb the running of the BCS, but whenever
an SEU is detected (most are flagged as a result of a checksum
calculation), the BCS microprocessor is rebooted. Occasionally,
several hours, or days of data have been lost before this is done.
2.7 Contact Persons
If you have any question about BCS software and/or instrument, or if you
need any advice in BCS data analyses, contact:
2.8
References
- ``BCS Experiment Users Guide", ed. K. J. H. Phillips.
- J. L. Culhane et al., ``The Bragg Crystal Spectrometer for Solar-A,"
1991, Sol. Phys. 136, 89.
- J. Lang et al., ``The Performance of the Bragg Crystal Spectrometer,"
1992, P.A.S.J. 44, 5, L55.
- J. Lang, et al., ``The Laboratory Calibration of the Yohkoh Bragg
Crystal Spectrometer," 1993, RAL Publication RAL-93-035.
- M. W. Trow, R. D. Bentley and J. L. Culhane, ``A Positional Sensitive
Proportional Counter for the Bragg Crystal Spectrometer on Solar-A,"
1989, SPIE 1159 EUV, X-ray and Gamma-Ray Instruments for Astronomy and
Atomic Physics, 252.
- M. W. Trow, A. C. Bento and A. Smith, ``Rate Dependent Image Distortions in
Proportional Counters," 1993, Nucl. Inst. & Meth. A, in press.
Converted at the YDAC on Oct 4, 2004
(from LaTEX using TTH, version 1.92, with postprocessing)